Photovoltaics Give Credibility To A Solar Revolution
Better and cheaper solar panels have led to rapid adoption of solar power. If progress continues, solar will not just address environmentalist goals, but also upset global energy geopolitics.
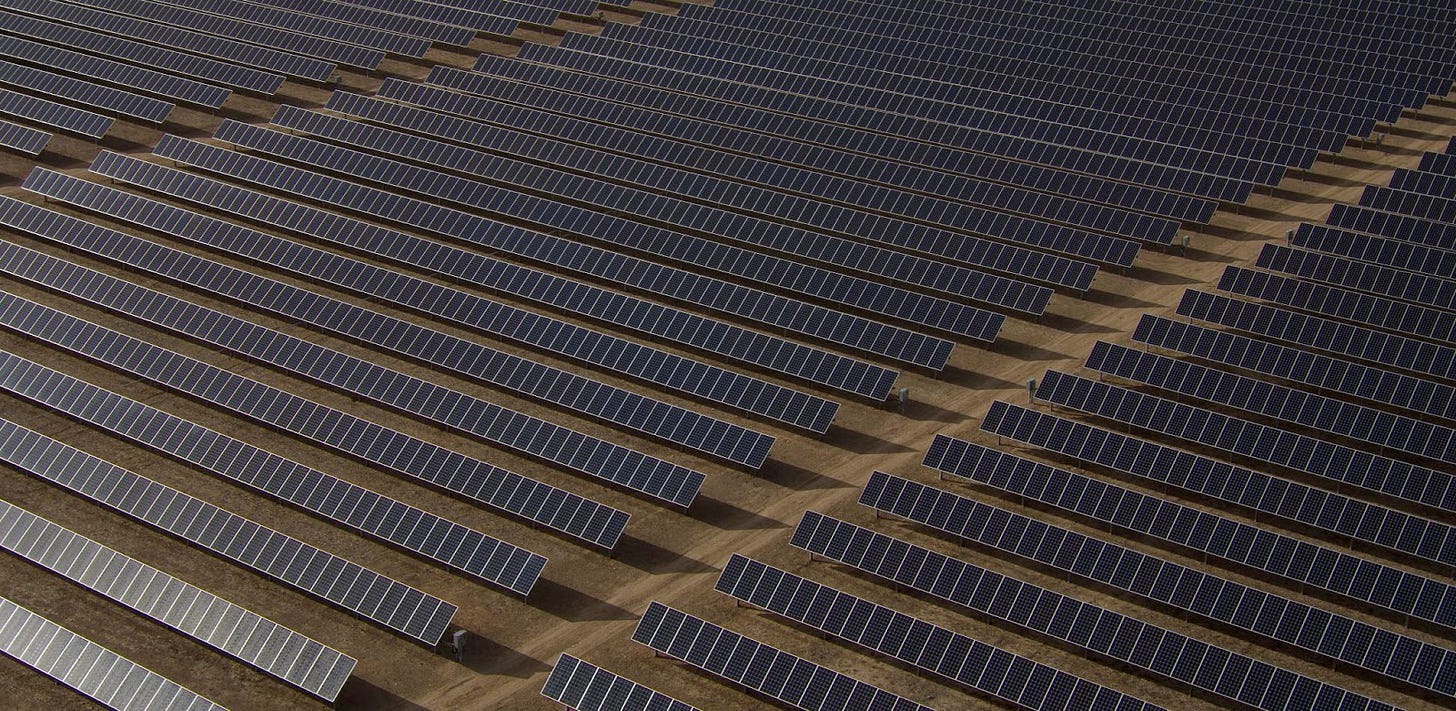
The total amount of solar energy that reaches the Earth’s surface each year is roughly 750,000 petawatt-hours (PWh), more than 5000 times global energy consumption in 2015.1 This abundance means there are few other sources of energy that could prove as disruptive to global energy markets and geopolitics as solar power. Since the year 2000, solar power has rapidly grown from a curiosity into a notable source of energy. From 2000 to 2020, global electricity consumption soared by 75% to 25.8 PWh and solar’s contribution grew 788-fold to 0.8 PWh—or 3.3% of all consumption.2 The price of solar power dropped dramatically from $359 per MWh to $37 per MWh between 2009 and 2020,3 and solar industry revenues soared to US$170 billion in 2020.4
You can listen to this Brief in full with the audio player below:
This progress has been driven by improvements in the efficiency and manufacturing of photovoltaics (PV), which make up 95% of the solar energy market.5 Photovoltaic systems combine several photovoltaic cells into a photovoltaic module—a solar panel—that generates power. They descend from the innovations of American engineer Russell Ohl,6 a researcher at AT&T’s Bell Labs, who filed his patent for a light-sensing device in 1941.7 In materials like silicon, absorbed light is directly converted into electricity as a result of the photovoltaic effect. There is also a much smaller market dedicated to concentrated solar thermal (CST) energy, which uses mirrors or lenses to concentrate sunlight onto a receiver to generate heat. But while CST has advantages for storing thermal energy, it can only be deployed at large scale and cannot be deployed in areas of low solar irradiation.
Solar power promises to be a critical part of the proposed transition to renewable energy championed by institutions in the developed world. While a transition to renewables is presented in the West as an environmental issue, any such transition from fossil fuels to an alternative—be it renewables, nuclear power, or even fusion—would both change the tax power of states as well as completely alter the balance of energy that underwrites international institutions. The growth of the photovoltaic industry thus has implications that go far beyond energy production. But rather than the West, it is China that is poised to accrue advantages from further progress in solar technology. China dominates the photovoltaic industry, controlling 79% of cell production in 2019.8 As has become common, Western institutions call for a future that is ultimately built in China.
Global Consequences of a Solar Revolution
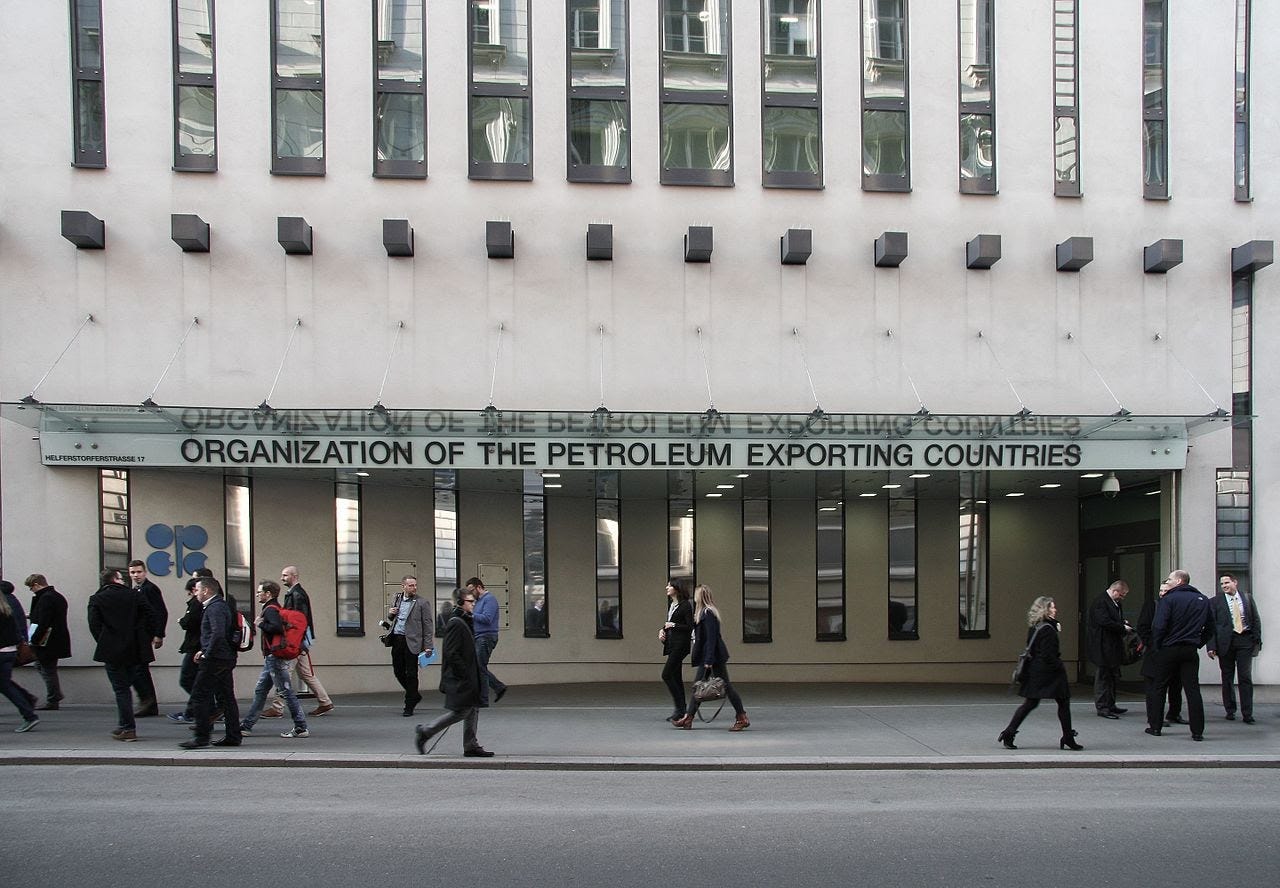
When it comes to energy, oil has been the most globalizing force in modern history, because oil is the easiest energy source to ship anywhere in the world. It makes the economics of importing energy less onerous, allowing development even in countries with few fossil fuel deposits. Though there still isn’t quite a true global market, changes in oil production in the Middle East matter for production and consumption as far away as China or America, giving rise to the political need of great powers to try and manage oil prices globally. The effect is particularly legible not just to states but to the population as well. By subsidizing low prices at the gas pump, many governments hope to avoid unrest. Oil-importing countries with stronger economies use taxes on this consumption as a way to raise their revenues.
If oil has a globalizing effect, natural gas has a regionalizing effect, as it is usually delivered through pipelines. In 2021, 46% of the European Union's total natural gas imports came from Russia.9 This dependency, especially of much wealthier Germany, has allowed Russia to use restrictions and threats of restrictions of natural gas export as an effective tool of foreign policy numerous times throughout the 2000s, while rewarding friendly client states with subsidized gas.10 The technology to liquefy natural gas then is a way to avoid such pressure, making gas more similar in its shipping constraints to oil. This means progress in such technology pushes towards globalization and weakens regional powers. A commonality that the oil and gas industries share is that they can generate great tax revenues even for states with low state capacity, allowing for militarily powerful and politically unified states with weak economies. States like Saudi Arabia, Iran, and Russia depend on their energy sector for both funding their armed forces and keeping the citizenry content. Compared to oil and natural gas, solar power is quite limited as a tactical weapon. If China were to congest the photovoltaic supply chain, there would be no immediate blackouts or even spikes in the price of electricity. It couldn’t use its leadership in manufacturing photovoltaics the way Russia can use gas or Saudi Arabia can use oil to achieve rapid realignment.
Coal, while it can be shipped and exported, is a localizing force. Since the beginning of the Industrial Revolution up to the present day, heavy industry clusters tightly around large coal deposits. The global effects of coal deposits are felt through the export of finished products, not directly through exporting energy. Much as exporting manufactured photovoltaics, manipulating exports of mined coal doesn’t lend itself to fruitful political use; however, the difference between manufacturing and mining is relevant. An exporter of photovoltaics such as Germany or China can carry out industrial policy aimed at growing their manufacturing sector at the expense of rivals. Their vendors can try to flood the market to crush competition and ensure the agglomeration benefits of production stay firmly with them for the foreseeable future. Finally, an important international investor like the United States or China can carry out the same kind of pressure they might with other infrastructure spending, by withholding solar projects in foreign jurisdictions. Cheaper and more abundant solar energy would likely be a localizing force that would drive demand for complements to solar’s intermittency—the fact that less power is generated in cloudy weather or at night—such as better batteries and natural gas.
“Dirty” coal and “clean” solar energy, however, have one final interesting connection. Since photovoltaics are a manufactured good, which consumes energy to manufacture, the energy mix of the producer becomes important for thinking about effects on carbon emissions. As China’s manufacturing output has soared from $625 billion in 2004 to $3.85 trillion in 2020, so has its reliance on cheap coal to power and meet those energy needs. Total coal generation rose from 13,155 TWh to 22,853 TWh from 2004 to 2020, with its share of energy reducing only mildly from 66% to 58%.11 When evaluating carbon emissions decreases touted in Western countries, it is important to note that those same countries have increasingly depended on China for imports of energy-intensive manufactured goods, including photovoltaics. From 2004 to 2020, U.S. imports from China doubled from about $200 billion to over $400 billion.12 European Union imports from China also doubled over the same period.13 For both the U.S. and European Union, the overwhelmingly dominant categories of imports from China in 2020 were manufactured goods like electronics and machinery. In 2020, the European Union imported €8 billion worth of solar panels, three-quarters of which came from China.14 Combined with the higher carbon cost of Chinese-made photovoltaics,15 it perhaps makes sense to view photovoltaics as a way of exporting the energy of coal burned here and now to consumers there and then. While this would undercut the environmentalist case for solar power, it would signify important changes for both the political economy of energy as well as global energy geopolitics.
The Production and Technology of Photovoltaics
Over 95% of the photovoltaics market is based on crystalline silicon wafers; the other 5% is accounted for by thin-film technologies, which are more flexible but less efficient. The crystalline market is divided between monocrystalline and multicrystalline cells. Due to improved manufacturing processes, the more efficient—but costly—monocrystalline silicon accounted for 80% of new photovoltaic installation capacity in 2020.16 The supply chain for photovoltaics is relatively simple. Silicon, the second most abundant element on the planet, is the key raw material. After being mined, this silicon has to be purified. Producing the very high purity silicon required for the photovoltaics industry (over 99.999%) has high technical requirements and is a comparatively profitable part of the supply chain. The result is polysilicon, the material upon which the photovoltaics industry is built.
Downstream from this is the ingot and wafer manufacturing process. An ingot is a cylinder of polysilicon. If this is a single crystal, it is called monocrystalline silicon. If it consists of multiple smaller crystals, it is multicrystalline silicon. Boron and phosphorus are then added in a “doping” process, creating n-type (negative) or p-type (positive) silicon. In highly automated workstations, ingots are then sliced into thin layers called wafers and polished. The next process is cell manufacturing. To create the cell, n-type and p-type wafers are layered together to create the p-n junction, converting light to electricity. This process is highly automated, but still price-sensitive to differences in wages. The final stage of manufacturing is module assembly. Cells are encapsulated in glass sheets to form modules and are cooked in laminating machines. Modules are assembled into arrays. The addition of specialist electrical equipment like inverters completes the system.17 Like the refining of silica into polysilicon, inverters are high-value relative to the middle manufacturing process. From here, the market moves to installation contractors, system integrators, and major utility companies; these vendors go on to manage the installed capacity of solar arrays.
The cost of photovoltaic hardware has declined, with the International Renewable Energy Agency (IRENA) claiming an 82% reduction in cost for solar photovoltaic panels from 2010 to 2019.18 This exceeds the cost reductions for onshore wind (40%) and offshore wind (29%) in the same period.19 This reduction was achieved through technology improvements and economies of scale in manufacturing processes, underwritten by Western government subsidies. Wafer surface areas increased while silicon thickness decreased. Most importantly, module efficiency improved from an average of 8% to 15% between 1980 and 2012, due to the advancements of American, German, and Japanese laboratories. Plant sizes increased and the cost of polysilicon dropped from $126/kg to $26/kg in the same period.20 The cost of non-silicon products involved in manufacturing, like glass and aluminum, was also critical. The locations responsible for this advancement were generally European, American, and Japanese. But critically, China’s contribution came with the further expansion of polysilicon and cell manufacturing capacity. In an industrial economy, the factory and the economies of scale it provides are the more important unit of innovation than the laboratory product.
When it comes to solar power, capacity and generation are two technical terms that need to be understood. Capacity, measured in watts (W), refers to the potential energy that can be generated under ideal conditions, defined as a clear bright day at high noon. Generation refers to the amount of energy produced over time and is measured in watt-hours (Wh). For example, if a solar array has a capacity of 1 GW (gigawatt), but is only operating at 20% efficiency, as is common, then it would produce 200 MWh (megawatt-hours) over one hour. Over 2 hours, this would translate into 400 MWh. When measured over all the hours in a year, this translates into trillions of watt-hours. In 2020, global capacity was 707.5 GW and photovoltaics globally generated 844.4 TWh of electricity.21 The theoretical global capacity factor—the percentage of potential energy that is generated by solar—is therefore 13.6%.22 At 100% efficiency, it should amount to a staggering 6.2 PWh, easily enough energy to power the United States for a year.
The capacity factor and energy payback time23 (EPBT) of photovoltaic systems are heavily dependent on the geographical location and the intensity of solar radiation, with sunnier areas being more ideal. Photovoltaic systems in northern Europe, therefore, have a lower potential capacity than systems near or south of the equator. This geographic limitation is challenging for the industry as the ideal areas for solar generation, like the central Asian steppe or the Sahel, generally lack proximity to modern energy infrastructure. According to the World Bank, 20% of the world’s population, dispersed across 70 countries, have excellent conditions for solar.24 These are almost all south of the equator and are disproportionately poor. All else being equal, advances in solar then provide an energy subsidy to developing economies.
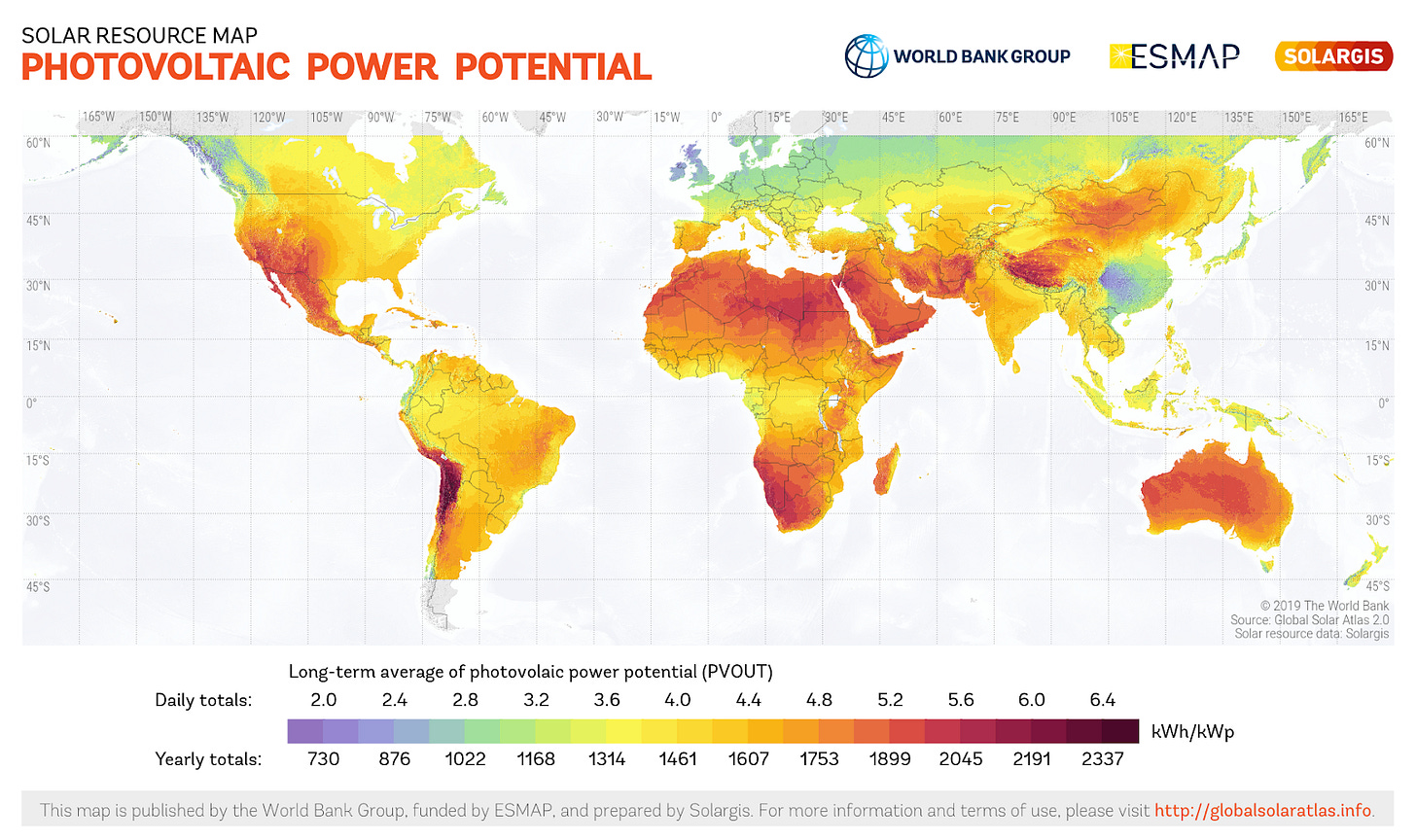
But for developed nations, price decreases for hardware are not translating to cost reductions for the wider grid. This is highlighted by the continual price increases of solar’s great champions, Germany25 and California.26 The capacity factor limitations of photovoltaics are well known and there is reason to believe that if energy share exceeds capacity factor, the costs of solar rise steeply.27 High penetration rates and low capacity factors combine to create an energy glut at the wrong time of the day. Solar capacity reduces electricity prices during the very hours when solar generators produce the most electricity. Beyond low levels of penetration, an increasing solar contribution results in lower average revenues per kW of installed solar capacity.28 When supporters focus on the levelized cost of energy (LCOE) and show solar and wind as the most competitive, they are not accounting for intermittency or grid integration. Levelized system cost of energy (LSCOE) and other metrics are being developed to highlight the diminishing value of intermittent renewables when their share of the grid supply aligns with their capacity factor.29
Advances and expansions in electrical grids to accommodate solar could help alleviate some of these issues. Maintenance costs can also be reduced through the introduction of automated cleaning processes like teleoperated robots.30 But the truly necessary innovations in solar rest with storage and cell efficiency. To solve intermittency, electricity storage through batteries is vital. China, for example, currently has 3 GW of installed capacity and is planning 30 GW by 2025 and 100 GW by 2030.31 For context, the International Energy Agency (IEA) projects a global need for 585 GW of installed energy storage capacity by 2030 to reach net-zero emissions by 2050. Importantly, subsidies in China’s solar industry have been scaled back and increased uncertainty about the state’s backing could make progress in this hugely ambitious rollout slower than hoped.32 As for batteries, the lithium-ion market is dominated by Chinese, Japanese, and South Korean companies. China’s leading player in this $27 billion market is CATL, with a 32.5% global market share.33 CATL recently expanded from supplying batteries to electric vehicles to developing lithium-centric energy storage.34 Their biggest announcement came through a joint venture with engineering company Fujian Yongfu.35
Crystalline silicon wafers are 95% of photovoltaic capacity, but due to their poor efficiency and slowing rate of improvement, researchers are developing non-crystalline alternatives. Defined by the U.S. National Renewable Energy Laboratory (NREL), these subcategories have either not been successfully commercialized, or have been limited to high-value applications. Thin-film solar is categorized as second-generation solar. Instead of silicon wafers, which can measure a thickness of up to 200 micrometers, the material is cut into thin film measuring down to several nanometers.36 This more flexible film can be integrated into infrastructure or used in consumer products like watches and pocket calculators. The record efficiency for these systems is 23.35%, as demonstrated by the Japanese firm Solar Frontier.37 Single-junction gallium arsenide (GaAs) is third-generation technology that could be applicable to thin-film wafers while enjoying a higher efficiency. Their record for efficiency is set at 29.1%, from American firm Alta Devices.38
Multi-junction cells are capable of absorbing different wavelengths of sunlight, making them much more efficient.39 The NREL holds the record of 47.1% lab efficiency, the record for all photovoltaic technologies.40 These cells hold huge promise due to their high efficiency, but their expense is considerable. They are commonly used in solar-powered space systems like the Mars Perseverance helicopters.41 There are other emerging photovoltaic technologies that have yet to mature. Perovskite-based solutions are the most prominent in this subcategory. Their record lab efficiency was recorded at 29.8% by Helmholtz-Zentrum Berlin in Germany.42 As can be seen in Figure 2, progress since 1976 has been substantive at the laboratory level, but scaling these alternatives to the level of crystalline silicon will test industrial capacity for any nation.
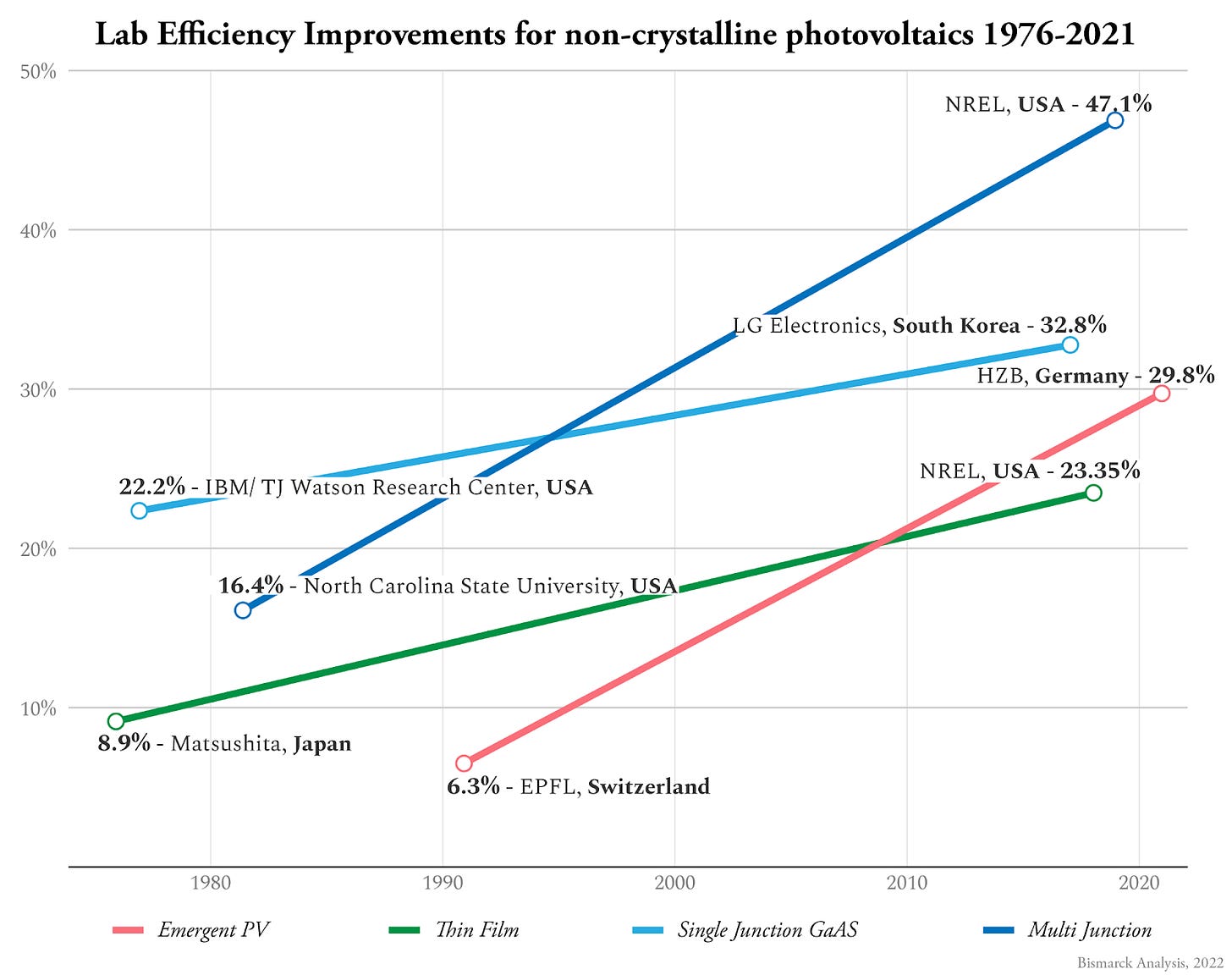
The benchmarks for cell efficiency are dominated by American research laboratories.43 This success in research mitigated concerns about losing manufacturing capacity for decades, both for solar and for technology more broadly. When listening to Democratic lawmakers concerned about offshoring in the 2000s, former U.S. Labor Secretary Robert Reich suggested “America’s going to do the design.” Certainly, if one were to observe breakthroughs and benchmarks for solar efficiency, the U.S. has continued to lead. Even with this dominance in official benchmarking, the competition from established Chinese solar companies has constricted R&D funding for alternative photovoltaics among Western companies, making commercialization very difficult. Given the difficulty of competing with China in the established photovoltaic market, other countries could dedicate long-term resources to commercializing technologies like thin-film and multi-junction photovoltaics.
China Captures and Bets on Solar
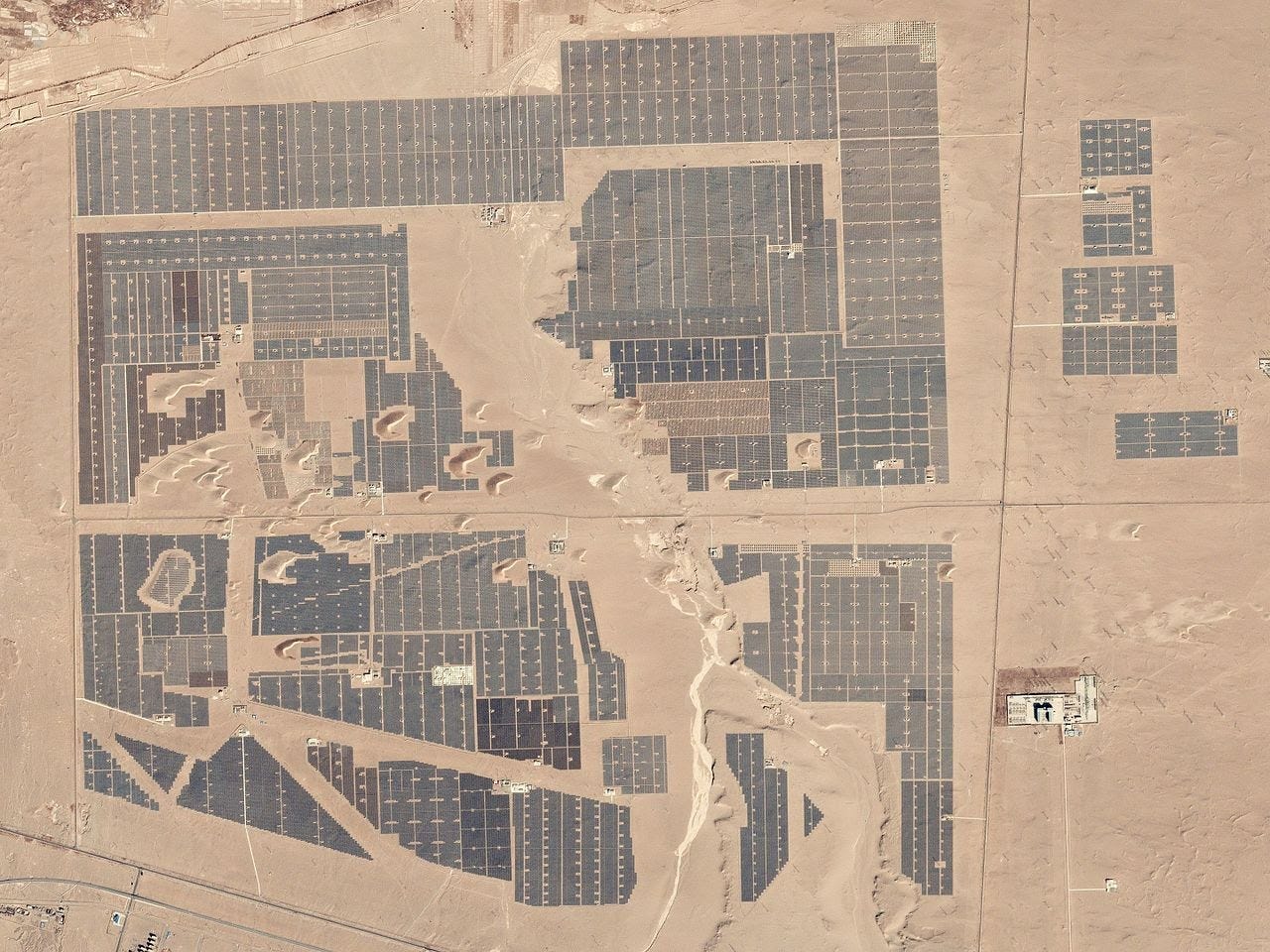
According to Bernreuter Research, Chinese firms controlled market share for polysilicon (87%), ingot (95%), wafer (97%), cell (79%), and module production (71%) in 2019.44 China is home to 261 manufacturing facilities for solar, with India coming a distant second at 51 and the U.S. has a mere 16.45 In 2020, 8 million metric tons of silicon were mined globally, of which slightly over two-thirds came from China’s western steppe.46 China’s polysilicon refineries are based in the hinterlands of Xinjiang, where there is cheap access to coal-fired electricity that was key to China’s ability to capture the solar supply chain.47 In 2020, 47% of global polysilicon was produced in Xinjiang.48 These historically poor territories are central to the photovoltaics supply chain, both for polysilicon refinement and for future deployments of larger installations due to high solar irradiation.49 Accusations of forced labor of China’s Uighur minority have been leveled by the EU and the U.S. and have been pushed by Western solar trade associations.50 Every step of photovoltaic production is consolidated by Chinese companies both on the mainland and in their overseas plants operating in Southeast Asia.
China is not only the largest manufacturer, but also the largest deployer of photovoltaics. In 2020, 261 TWh of solar energy were generated in China, while 154 TWh were generated in Europe and the United States generated 133 TWh.51 From 2015 to 2020, global solar capacity increased from 40 GW to 707 GW (+1,663%). For China, the growth over the same five years was from 1 GW to 254 GW (+24,742%).52 In 2020, China got 3.42% of its electricity production from solar, slightly higher than the world average of 3.27%.53 Though still a priority, photovoltaics are not favored over other “clean” power sources. Unlike in California and Germany, China’s deployment of photovoltaics does not come at the expense of nuclear energy, where $440 billion has been earmarked for investments up to 2035.54 Nevertheless, government documents and sponsored research envision an accelerated expansion of solar, emphasizing deployments in China’s western provinces. While far less ambitious than environmentalist goals to eradicate fossil fuel use, the 2021 fourteenth five-year plan (FYP) commits China to have non-fossil fuels account for 20% of energy use by 2025, compared to 15% at the end of 2019.55 The country also aims to see energy demand peak in 2035.56 This is noteworthy in that China, at least officially, does not deviate from the consensus view that reducing energy consumption per capita is necessary in the medium term.
Another key component of the fourteenth FYP emphasizes the consolidation of industries into powerful conglomerates. This is seen in the establishment of a massive rare-earth metal conglomerate.57 Thirty percent of this new company, China Rare Earth Metal Corp., will be owned by China’s State-owned Assets Supervision and Administration Commission (SASAC).58 Aside from the state-owned utilities, China’s solar manufacturing market is nominally private, but companies have shareholders that have direct relationships with the government. This gives them security, but serves as a form of indirect control. Given the direction of the latest FYP, there is reason to believe the implicit control the Chinese government has on its solar industry will eventually become explicit, possibly through the creation of conglomerates for different parts of the supply chain.
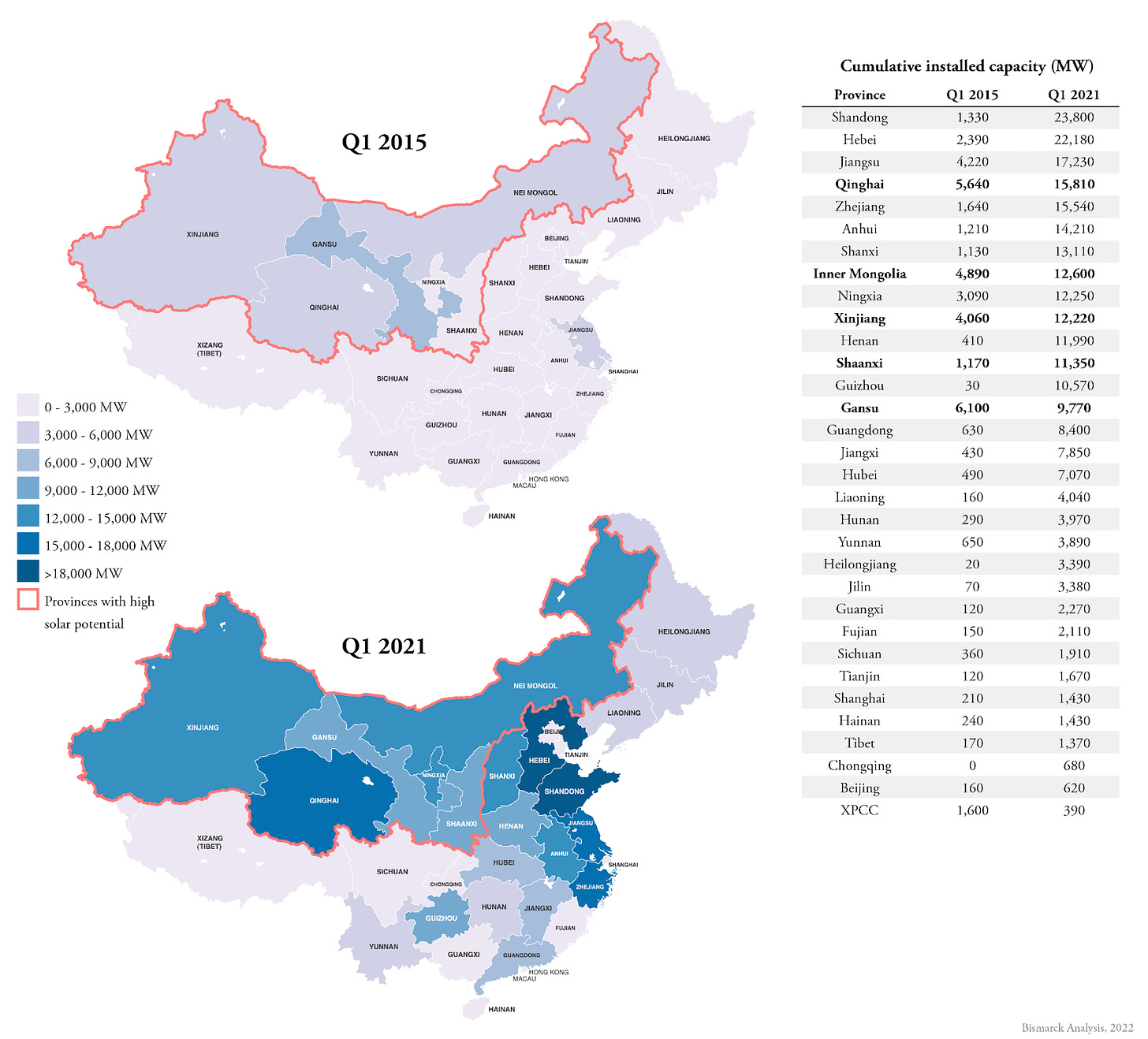
To date, China’s deployment of photovoltaics has concentrated on the country’s heavily populated eastern coastline. There is relatively modest deployment in the western provinces, despite these being the areas where the highest efficiency can be attained due to climate and solar irradiation. The challenge is a lack of grid infrastructure to transport electricity over long distances, which forces solar installations to be located eastward. To solve this, the government is undertaking a massive expansion in ultra-high-voltage direct current (UHVDC) transmission lines.59 The clearest example of this is the Changji-Guquan UHVDC link which was commissioned in 2019. It stretches 3000 km, has a voltage of 1100 kilovolts, and has a capacity of 12 GW. This line can power 50 million homes in eastern China yearly with solar power from the west. This project cost $4.7 billion, and more lines are currently being constructed. Achieving this on a larger scale and building out photovoltaics to their maximum potential in the west of China would require hundreds of billions of dollars.
Germany and California Become Consumers Rather Than Creators of a Solar Future
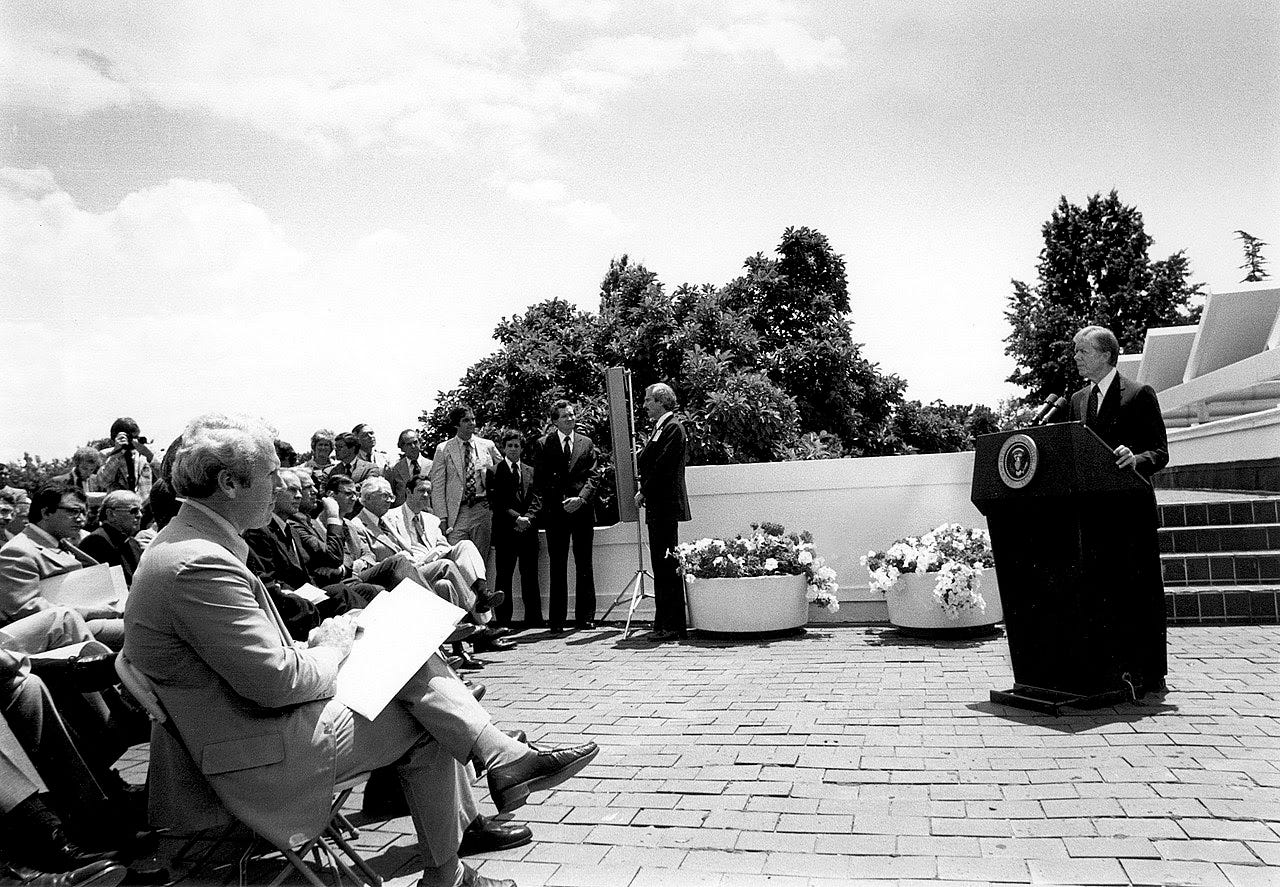
How this consolidation came about makes for an interesting study of how institutions succeed or fail at driving technological progress. Following the 1973 oil shock caused by OPEC’s infamous oil embargo, energy consumption in the developed world began to stagnate and more attention was paid to energy security.60 Before this, growing energy consumption was considered the hallmark and even driver of technological progress. After the oil shock, high energy consumption came to be seen as a political vulnerability. While nuclear energy had enjoyed promising growth up to this point, concerns around safety and weapons proliferation were growing and, in the eyes of activists, vindicated by accidents at Three Mile Island in 1979 and Chernobyl in 1986. Prominent environmentalists like David Brower and the physicist and writer Amory Lovins criticized centralized “hard energy” from nuclear and fossil fuels and instead touted “soft” distributed energy based on efficiency and lowering consumption.61 Lovins in 1976 championed a transition towards “soft” renewables and a gradual reduction in energy consumption.62 While concerns about climate change were evident, Lovins suggested that coal use continue, while nuclear fission be phased out immediately. This vision for efficiency, decentralization, and eventually reducing consumption gained support quickly; U.S. President Jimmy Carter installed solar panels on the White House in 1977 as a gesture of approval.63
By the 1990s, Lovins’ vision had gained considerable support across the developed world. This was particularly the case in Germany, whose government awarded Lovins the Order of Merit in 2016 for his intellectual underwriting of Germany’s Energiewende (lit. “energy turnaround”)—Germany’s long-running plan to transition from nuclear and fossil fuels to renewables, in particular wind, hydro, and solar.64 Growing concerns about global warming and climate change caused by carbon dioxide emissions from using fossil fuels further strengthened the environmentalist case for solar energy. Climate change came to be considered a justification for a different kind of globalization than the one produced by the U.S.-centered petrodollar. The success of the Montreal Protocol, signed in 1987 to limit emissions of ozone-depleting substances, lent high hopes to the idea of limiting global carbon emissions.65 Since global limits on carbon emissions would effectively function as global limits on economic growth, this would favor incumbents in the developed world.
These ideals came to drive policy in countries that might stand to benefit from such alternative globalization. The photovoltaics market grew considerably with the popular adoption of feed-in tariffs (FITs) by the German government in 2000.66 FITs are designed to subsidize producers of renewable energy with a cost-based, rather than demand-based, price for the electricity they provide to the grid. This offers price certainty and is guaranteed by long-term contracts, thereby reducing the risk of investment. These subsidies accelerated photovoltaic installations and led to strong solar company ecosystems in Germany and Japan. Meanwhile, Chinese interest was marginal and tied to bringing electricity to poor rural areas, through the Brightness Rural Electrification Program. This project, started in 1998, was budgeted at $50 million and was designed to provide renewable energy to 23 million people in China’s impoverished western provinces.67 These programs, perhaps modeled after German and Japanese ones, were too modest to make much of a difference.
But as developed economies continued to pursue solar subsidies as a means of developing their domestic ecosystem, Chinese entrepreneurs saw the potential for a new export market they could disrupt. While photovoltaics did not gain central government support in China until 2009, local governments began considerable subsidies in 2001, and further capital was provided by foreign direct investment and American private equity markets.68 The genesis of Chinese photovoltaics involved enterprising local government, capable researchers, and a reliance on research infrastructure in the developed world. Zhengrong Shi, for example, was a Chinese researcher who was mentored by renowned Australian engineering professor Martin Green. In 2000, Shi had a fateful meeting with local government officials from Jiangsu. They offered to fund a new solar photovoltaics company back in China with Shi at the helm.69 This birthed Suntech, which quickly became China’s largest photovoltaics producer. Domestic interest was minimal, but German demand was enough to kickstart the company. Suntech’s success brought in new interest from entrepreneurs and local governments. The latter subsidized domestic demand and supply, incorporating FITs to stimulate deployment. On the supply side, local development finance institutions made cheap credit available, while state-owned entities (SOEs) subsidized early adopters.70
While Chinese dynamism and expertise were evident in the success of their solar industry, German demand and American capital were key. Early Chinese vendors, including Suntech, were funded by American entities like Goldman Sachs and listed on American stock exchanges. Initially, China targeted the comparatively low-value manufacturing process for solar cells and modules. This was achieved by buying German machine tools and hiring Western consultants. This transfer of photovoltaics manufacturing equipment and institutional knowledge was critical. Even throughout the 2000s, Western governments restricted technology transfers for what they considered to be mature and security-sensitive sectors like aerospace and semiconductors. They didn’t anticipate China competing in a relatively complicated high-value industry. The dominance in manufacturing was complemented by Chinese firms entering the more high-value parts of the process, including polysilicon refinement and inverters. The then-common belief that China’s comparative advantage and economic strategy would be limited to low-value manufacturing proved false.
The transformation was rapid. As early as 2007, China had become the single largest producer of photovoltaic cells, with 28% of global production.71 This takeover has been a huge stimulant to the Chinese labor market. By 2019, China’s solar industry employed 4.57 million people.72 In contrast, the U.S. supported just 231,000 solar jobs, far below the five million promised by President Barack Obama in 2008.73 There was a belated American attempt at protectionism by deploying tariffs as high as 249% on photovoltaic cells in 2012.74 China responded through retaliatory tariffs and vendors moving production to other low-wage countries like Vietnam and Malaysia.75 The tariffs were targeted towards polysilicon refinement, where American production never recovered. For remaining American refiners like Hemlock, Chinese tariffs still stand at 55% and have been renewed until 2025.76
The European Union similarly placed tariffs on Chinese-produced modules on anti-dumping grounds in 2013,77 but these punitive measures were opposed by the German government, proved ineffective, and were lifted in 2018.78 On trade, the U.S. and Europe are critically misaligned. The U.S. has a trade as a percentage of GDP ratio of 23% and is well-placed for trade wars. While the EU only has a ratio of 26%, powerful individual nations like Germany are reliant on future increases in trade with China to supplement the diminishing returns they get from intra-European trade.79 Misalignment of interests exists between industry sectors as well as between countries. SolarWorld, a German manufacturer, supported EU tariffs, while the German automotive industry and solar trade associations disapproved, arguing deployment was more important than the source.80
While state support was critical, dominance was not planned or strategized from the top down. Success at the entrepreneurial level, in collaboration with local governments, preceded central government interest. It was only in 2010 that solar energy was earmarked as a key “strategic emerging industry” (SEI) by China’s State Council and central government support grew. The China Development Bank (CDB) provided direct loans to solar companies, including $47 billion made available between 2010 and 2011, as Chinese market share jumped from 48% to 59%.81 Subsidies allowed photovoltaics manufacturers to sustain enormous losses as global competitors folded or cut back their research budgets. The median operating margin for a group of Chinese manufacturers tracked by the NREL fell to negative 40% in 2011, recovered briefly, and fell again to negative 30% in 2012.82 Chinese manufacturers had comparatively high debt-to-capital ratios.83 While Suntech went into bankruptcy, the Chinese photovoltaics industry as a whole was more resilient than any other market.
By 2012, the solar industry was undeniably dominated by China. The established wisdom is that China is adept at copying Western technology successes. The more nuanced reality is that China remains heavily reliant on foreign vendors for high-value, mature industries like semiconductors, machine tools, transformer-converters, and aircraft. Where China has outperformed other countries is in capturing nascent and fragile industries, including photovoltaics. In the early days, the photovoltaics market was unguarded from new entrants and poor coordination between competitors was critical. Similar stories of Chinese dominance in nascent technology markets include consumer and commercial drones, via the dominance of DJI, and the Chinese monopoly on application-specific integrated circuits (ASICs) for cryptocurrency mining. Much like for photovoltaics, Chinese drone developers relied on industrial espionage of American technology, built their own prototypes, engaged in fierce internal competition, had centralized operations with management and manufacturing in the same jurisdictions, attained government subsidies, maintained high-quality standards, and promoted export-led growth. China’s entrepreneurial class is quick in developing new markets. Suntech’s success in 2001 spurred on others, who have since gone on to take over the market. China now has a new class of billionaires related to solar panels.84 While both China and the United States publish ambitious policy documents about building the future, only one system is optimized for such undertakings.
Incumbents wanted climate neutrality without an energy revolution
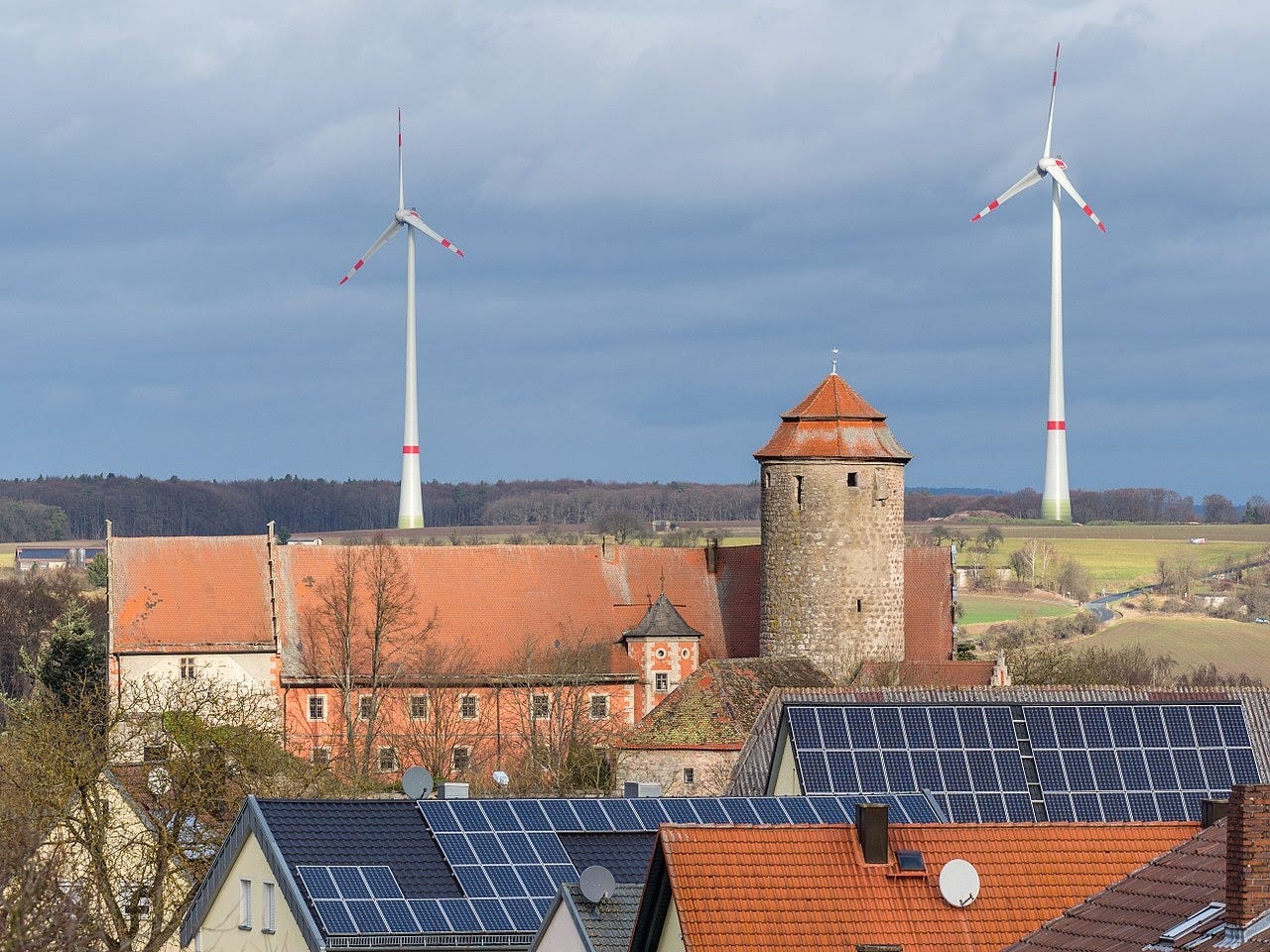
In 2019, the European Union announced a “European Green Deal” aiming to reach net-zero emissions of greenhouse gases across the bloc by 2050 and appointed long-time Eurocrat Frans Timmermans in a new executive position to spearhead this strategy.85 Similarly, the United Nations officially aims to “increase substantially” the global share of renewable energy usage by 2030, under Sustainable Development Goal 7, and uses language in support of net-zero emissions by 2050.86 In late 2021, U.S. President Joe Biden’s administration announced a plan to supply almost half of the United States’ electricity with solar power by 2050, in line with a campaign promise to reach net-zero emissions by 2035.87 But while photovoltaics are among the only technologies that could deliver on these long-term plans proposed by Western institutions and governments, photovoltaics remain overwhelmingly built in China. From the mining of raw materials to final assembly, Chinese companies dominate every step of the production of photovoltaics.
In the West, the objectives of the renewable energy transition are attempts to reduce negative externalities, rather than attempts to significantly increase energy production and consumption. In Germany, for instance, the energy transition has determined industrial policy even as manufacturing output has stagnated since 2008.88 But for fast-growing industrial nations looking to significantly increase energy consumption, renewables are simply another plank of a strategy for energy abundance that can be employed if the economics make sense. The prospect of solar energy emerged in the West as an alternative to the perceived dangers of nuclear and fossil fuel energy. In China, both solar and nuclear continue to be pursued simultaneously. China’s pursuit of industrial and energy growth has, perhaps counterintuitively, proven to be more successful at building out alternatives to fossil fuels than the environmentalist approach. This success was not predicted by Western institutions; the IEA for example consistently underestimated the rate at which new photovoltaic installations would be added from 2006 to 2018.89
Global coordination to reduce carbon emissions—exemplified by agreements such as the 1997 Kyoto Protocol or the 2016 Paris Agreement—has largely failed90 and will most likely continue to fail for fundamental reasons of the political economy of energy. Global coordination for low energy consumption is self-defeating since it rests on Western dominance, which itself rests on oil-backed globalization. Low energy consumption in one country, as practiced by Germany for example, is a self-limiting strategy. The carbon not emitted and cheap energy not tapped will instead be emitted and tapped by new industrial giants such as China and, eventually, India. Rather, localized and abundant solar energy seems to be one of the only politically viable solutions. Localizing energy markets would help break international coordination based on oil and gas. If those local solar markets achieve electricity too cheap to meter, as nuclear once promised to do, the market would become an enemy, rather than a friend, to the coal industry. This approach isn’t favored in developed countries because it would disrupt current political patronage in a way that promises of energy stagnation do not. Moreover, it fails to satisfy environmentalists and skeptics of technology, because although it would solve the problem of carbon emissions-driven climate change, it doesn’t resolve any of the other environmental91 or social92 problems of industrial society. The question then is whether any live players can change the developed world’s approach to energy after 50 years on a road to nowhere.
Vaclav Smil “Energy: A Beginner's Guide”, (OneWorld Press), 2017, p. 32.
Hannah Ritchie and Max Roser, “Renewable Energy,” Our World In Data, 2020, https://ourworldindata.org/renewable-energy#solar-energy-generation
“Lazard’s Levelized Cost of Energy Analysis — Version 14.0”, Lazard, October 2020, https://www.lazard.com/media/451419/lazards-levelized-cost-of-energy-version-140.pdf
“Solar Power Market Size, Share and COVID-19 Impact Analysis (...)” Fortune Business Insights, April 2021, https://www.fortunebusinessinsights.com/industry-reports/solar-power-market-100764
“Photovoltaics Report 2021,” Fraunhofer Institute, July 27, 2021 https://www.ise.fraunhofer.de/content/dam/ise/de/documents/publications/studies/Photovoltaics-Report.pdf
“Obituary for Russell Shoemaker Ohl (Aged 89),” The Morning Call, October 16, 1987, https://www.newspapers.com/clip/48036981/obituary-for-russell-shoemaker-ohl/
The patent can be viewed at: https://patentimages.storage.googleapis.com/d3/8b/71/374769ceefb2fa/US2402662.pdf
Johannes Bernreuter, “Solar Value Chain,” Bernreuter Research, June 29, 2020, https://www.bernreuter.com/solar-industry/value-chain/
“EU Imports Of Energy Products - Recent Developments,” Eurostat, October 2021, https://ec.europa.eu/eurostat/statistics-explained/index.php?title=EU_imports_of_energy_products_-_recent_developments#Main_suppliers_of_natural_gas_and_petroleum_oils_to_the_EU
For a review of Russian energy diplomacy during the period 2000-2010, see Randall Newnham (2011). Oil, Carrots, and Sticks: Russia’s Energy Resources as a Foreign Policy Tool. Journal of Eurasian Studies 2(2), 0–143. doi:10.1016/j.euras.2011.03.004; For more recent instances of Russian coercive energy diplomacy, see Gabriel Collins, “Russia’s Use of the ‘Energy Weapon’ in Europe,” Baker Institute for Public Policy Brief, August 18, 2017, https://www.bakerinstitute.org/media/files/files/ac785a2b/BI-Brief-071817-CES_Russia1.pdf
Hannah Ritchie and Max Roser, “Fossil Fuels,” Our World In Data, 2020, https://ourworldindata.org/fossil-fuels
“United States Imports from China,” TradingEconomics, accessed January 23, 2022, https://tradingeconomics.com/united-states/imports/china
“European Union Imports from China,” TradingEconomics, accessed January 23, 2022, https://tradingeconomics.com/european-union/imports/china
Max Hall, “Eurostat: EU Had €6.2 Billion Solar Panel Trade Deficit In 2020,” PV Magazine, December 6, 2021, https://www.pv-magazine.com/2021/12/06/eurostat-eu-had-e6-2-billion-solar-panel-trade-deficit-in-2020/
Ian Clover, “Chinese-Made Panels Have Twice The Carbon Footprint Of European Ones, Study Finds,” PV Magazine, June 2, 2014, https://www.pv-magazine.com/2014/06/02/chinese-made-panels-have-twice-the-carbon-footprint-of-european-ones-study-finds_100015278/
“Photovoltaics Report 2021”, Fraunhofer Institute, July 27, 2021 https://www.ise.fraunhofer.de/content/dam/ise/de/documents/publications/studies/Photovoltaics-Report.pdf
Radomir Tylecote, “Ownership and Innovation in Chinese Solar Photovoltaic Firms: An Analysis of the Effects of State, Private, and Foreign Shareholding On Patenting Performance,” Imperial College London, 2015 https://spiral.imperial.ac.uk/handle/10044/1/26286
Molly Lempriere, “Solar Photovoltaics Costs Fall 82% Over The Last Decade, Says IRENA,” Solar Power Portal, June 3, 2020, https://www.solarpowerportal.co.uk/news/solar_pv_costs_fall_82_over_the_last_decade_says_irena
Ibid.
Goksin Kavlak, James McNerney, Jessika E. Trancik, “Evaluating the Causes of Cost Reduction in Photovoltaic Modules”, MIT, Energy Policy, 123(2018), 700–710.
Hannah Ritchie and Max Roser, “Renewable Energy,” Our World In Data, 2020, https://ourworldindata.org/fossil-fuels
Ibid.
EPBT is the time it takes for the deployment to produce energy equal to the energy it consumes over its lifetime, including sourcing, manufacture, installation, maintenance and disposal.
“Global Photovoltaic Power Potential By Country,” World Bank Energy Sector Management Assistance Program, June 2020, https://documents1.worldbank.org/curated/en/466331592817725242/pdf/Global-Photovoltaic-Power-Potential-by-Country.pdf
“Electricity Prices for Household Consumers, First Half 2021,” Eurostat, 2021, https://ec.europa.eu/eurostat/statistics-explained/index.php?title=File:Electricity_prices_for_household_consumers,_first_half_2021_v5.png
“Electric Power Monthly,” EIA, October 2021, https://www.eia.gov/electricity/monthly/epm_table_grapher.php?t=epmt_5_6_a
David Roberts, “The Economic Limitations of Wind and Solar Power”, Vox, April 2015, https://www.vox.com/2015/6/24/8837293/economic-limitations-wind-solar
J.M. Korhonen, “Graphic of the Week: Having Too Much and Too Little Renewables – At The Same Time,” The unpublished notebooks of J. M. Korhonen, April 2014, https://jmkorhonen.net/2014/04/08/graphic-of-the-week-having-too-much-and-too-little-renewables-at-the-same-time/
Falko Ueckerdt, Lion Hirth, Gunnar Luderer, Ottmar Edenhofer, “System LCOE: What are the Costs of Variable Renewables?” Energy, 63 (2013), 61–75.
Julia Mericle, “RE2 Robotics Receives Funding to Develop Robots for Solar Field Construction,”Pittsburgh Business Times, March 2, 2021, https://www.bizjournals.com/pittsburgh/news/2021/03/02/re2-robotics-receives-funding-to-develop-for-solar.html
“Energy Storage Tracking Report,” IEA, November 2021, https://www.iea.org/reports/energy-storage
Eric Ng, “As China Moves to Reduce Subsidy Load, Uncertainties Mount for Country’s Wind And Solar Energy Sector,” South China Morning Post, March 2021, https://www.scmp.com/business/companies/article/3124106/china-moves-reduce-subsidy-load-uncertainties-mount-countrys
Bruno Venditti, “Electrification Ranked: The Top 10 EV Battery Manufacturers,” Visual Capitalist, September 25th 2021, https://elements.visualcapitalist.com/ranked-top-10-ev-battery-makers/
“China’s CATL Expands Energy Storage Business,” Argus Media, October 25, 2021, https://www.argusmedia.com/en/news/2266896-chinas-catl-expands-energy-storage-business
“CATL Joins Forces with Fujian Yongfu to Establish JV Firm,” CATL Website, February 6, 2021, https://www.catl.com/en/news/639.html
The material for thin-film is generally one of three options; amorphous silicon, cadmium telluride or Copper indium gallium selenide.
Mark Hutchins, “Solar Frontier Hits New CIS Cell Efficiency Record,” PV Magazine, January 21, 2019, https://www.pv-magazine.com/2019/01/21/solar-frontier-hits-new-cis-cell-efficiency-record/
“Alta Sets Flexible Solar Record with 29.1% GaAs Cell,” Optics.org, December 12, 2018, https://www.optics.org/news/9/12/19
“Multijunction III-V Photovoltaics Research,” U.S. Office of Energy Efficiency and Renewable Energy, 2020, https://www.energy.gov/eere/solar/multijunction-iii-v-photovoltaics-research
“News Release: NREL Six-Junction Solar Cell Sets Two World Records for Efficiency,” NREL, April 13, 2020, https://www.nrel.gov/news/press/2020/nrel-six-junction-solar-cell-sets-two-world-records-for-efficiency.html
“Photovoltaics,” Spectrolabs, 2021 https://www.spectrolab.com/photovoltaics.html
“World Record Again at Hzb: Almost 30 % Efficiency for Next-Generation Tandem Solar Cells,” Helmholz-Zentrum Berlin, November 22, 2021, https://www.helmholtz-berlin.de/pubbin/news_seite?nid=23248;sprache=en
“Best Research-Cell Efficiency Chart,” NREL, 2021 https://www.nrel.gov/pv/cell-efficiency.html
Johannes Bernreuter, “Solar Value Chain,” Bernreuter Research, June 29th 2020, https://www.bernreuter.com/solar-industry/value-chain/
Jennifer A Dlouhy, “How China Beat the U.S. to Become World's Undisputed Solar Champion,”Bloomberg, June 4, 2021, https://www.bloomberg.com/news/articles/2021-06-04/solar-jobs-2021-how-china-beat-u-s-to-become-world-s-solar-champion?sref=ZqW0mZJf
“Silicon Statistics and Information”, USGS, 2020 https://pubs.usgs.gov/periodicals/mcs2021/mcs2021-silicon.pdf
Matthew Dalton, “Behind the Rise of U.S. Solar Power, a Mountain of Chinese Coal,” The Wall Street Journal, July 31, 2021, https://www.wsj.com/articles/behind-the-rise-of-u-s-solar-power-a-mountain-of-chinese-coal-11627734770
David Feldman, Kevin Wu, Robert Margolis, “H1 2021 Solar Industry Update,” NREL, 2021, https://www.nrel.gov/docs/fy21osti/80427.pdf
Luka Jukic, “Why China Will Decide the Future of the Steppe,” Palladium Magazine, August 8, 2020, https://palladiummag.com/2020/08/08/why-china-will-decide-the-future-of-the-steppe/
Aitor Hernández-Morales. “Fears Over China’s Muslim Forced Labor Loom Over Eu Solar Power,” February 10, 2021, https://www.politico.eu/article/xinjiang-china-polysilicon-solar-energy-europe/
Hannah Ritchie and Max Roser, “Solar Power Generation,” Our World In Data, 2020, https://ourworldindata.org/grapher/solar-energy-consumption?tab=table
Hannah Ritchie and Max Roser, “Renewable Energy,” Our World In Data, 2020, https://ourworldindata.org/renewable-energy#solar-energy-generation
Hannah Ritchie and Max Roser, “Renewable Energy,” Our World In Data, 2020, https://ourworldindata.org/renewable-energy#solar-in-the-energy-and-electricity-mix
Dan Murtaugh and Krystal Chia, “China’s Climate Goals Hinge on a $440 Billion Nuclear Buildout,” Bloomberg, November 2, 2021, https://www.bloomberg.com/news/features/2021-11-02/china-climate-goals-hinge-on-440-billion-nuclear-power-plan-to-rival-u-s
Benjamin Cooper “China’s 14th Five-Year Plan (2021-2025) Report,” Hill & Knowlton Strategies, April 1, 2021, https://www.hkstrategies.com/en/chinas-14th-five-year-plan-2021-2025-report/
“Full slide from Professor He Jiankun's presentation,” Tsinghua University, October 19, 2020, https://mp.weixin.qq.com/s/S_8ajdq963YL7X3sRJSWGg
Shunsuke Tabeta, “China to Create Rare-Earths Giant by Joining Three State Companies,” Asia Nikkei, October 24, 2021, https://asia.nikkei.com/Economy/China-to-create-rare-earths-giant-by-joining-three-state-companies
“China to Launch State-Owned Rare Earth Company,” Mining Technology, December 23, 2021, https://www.mining-technology.com/news/china-rare-earth-company/
Mark Champion, “The Future of Power Is Transcontinental, Submarine Supergrids,” Bloomberg, June 9, 2021, https://www.bloomberg.com/news/features/2021-06-09/future-of-world-energy-lies-in-uhvdc-transmission-lines?sref=ZqW0mZJf
Jason Crawford, “Progress, Stagnation, and Flying Cars,” Roots of Progress, November 6, 2020 https://rootsofprogress.org/where-is-my-flying-car
Amory Lovins, “Energy Strategy: The Road Not Taken,” Foreign Policy, 1976, https://rmi.org/insight/energy-strategy-the-road-not-taken/
Ibid.
Joshua Green, ‘The Elusive Green Economy, The Atlantic, July 2009, https://www.theatlantic.com/magazine/archive/2009/07/the-elusive-green-economy/307554/
Christian Roselund, “Amory Lovins Awarded the German Order of Merit for His Vision of the Energy Transition,” PV Magazine, March 19, 2016, https://www.pv-magazine.com/2016/03/19/amory-lovins-awarded-the-german-order-of-merit-for-his-vision-of-the-energy-transition_100023804/
McKenzie, R., Bernhard, G., Liley, B. et al. Success of Montreal Protocol Demonstrated by Comparing High-Quality UV Measurements with “World Avoided” Calculations from Two Chemistry-Climate Models. Sci Rep 9, 12332 (2019). https://doi.org/10.1038/s41598-019-48625-z
“The German Feed-in Tariff,” Future Policy, accessed January 25, 2022, https://www.futurepolicy.org/climate-stability/renewable-energies/the-german-feed-in-tariff/
“Renewable Energy in China,” NREL, April, 2004, https://www.nrel.gov/docs/fy04osti/35790.pdf
Donald Chung, Kelsey Horowitz, and Parthiv Kurup, “Emerging Opportunities and Challenges in U.S. Solar Manufacturing,” NREL, May 2016, https://www.nrel.gov/docs/fy16osti/65788.pdf
Royce Kurmelovs, “‘Insanely Cheap Energy’: How Solar Power Continues to Shock the World,” The Guardian, April 24, 2021, https://www.theguardian.com/australia-news/2021/apr/25/insanely-cheap-energy-how-solar-power-continues-to-shock-the-world
“Industrial Policy, Trade, and Clean Energy Supply Chains,” CSIS, February 2021, https://csis-website-prod.s3.amazonaws.com/s3fs-public/publication/210224_Ladislaw_Industrial_Policy.pdf?DRja.V6axwyBE_photovoltaics6Chmdi5k2VqOq33n
David M. Hart, “ The Impact of China’s Production Surge on Innovation in the Global Solar Photovoltaics Industry,” ITIF, October 5, 2020, https://itif.org/publications/2020/10/05/impact-chinas-production-surge-innovation-global-solar-photovoltaics
Bingchun Liu, Chengyuan Song, Qingshan Wang & Yuan Wang, “ Forecasting of China’s Solar Photovoltaics Industry Installed Capacity and Analyzing of Employment Effect: Based on GRA-Bilstm Model,” Environmental Science and Pollution Research (2021), https://link.springer.com/article/10.1007/s11356-021-15957-1
US Department of Energy, “Solar Futures Study,” September 2021, https://www.energy.gov/sites/default/files/2021-09/Solar%20Futures%20Study.pdf
Suzanne Goldenberg, “US Commerce Department Brings Heavy Tariffs Against Chinese Solar Panels,” The Guardian, May 17, 2012, https://www.theguardian.com/world/2012/may/17/us-tariffs-chinese-solar-panels
Jennifer A Dlouhy, “How China Beat the U.S. to Become World's Undisputed Solar Champion,” Bloomberg, June 4, 2021, https://www.bloomberg.com/news/articles/2021-06-04/solar-jobs-2021-how-china-beat-u-s-to-become-world-s-solar-champion?sref=ZqW0mZJf
Johannes Bernreuter, “New Chinese Polysilicon Duties are Slap in the Face to Trade Deal,” Bernreuter Research, January 19, 2020, https://www.bernreuter.com/newsroom/polysilicon-news/article/new-chinese-polysilicon-duties-are-slap-in-the-face-to-u-s-trade-deal/
Robin Emmott, “EU, China Resolve Solar Dispute - Their Biggest Trade Row by Far,“ Reuters News, July 27, 2013, https://www.reuters.com/article/us-eu-china-solar-idUSBRE96Q03Z20130727
Keith Bradsher and Melissa Eddy, “China Divides Europe in Fight Against Tariffs,” The New York Times, May 28, 2013, https://www.nytimes.com/2013/05/29/business/global/china-divides-eu-in-fight-against-tariffs.html
“Trade as Percentage of GDP,” World Bank Data, 2020 https://data.worldbank.org/indicator/NE.TRD.GNFS.ZS?locations=EU-DE-FR-CN-JP&most_recent_value_desc=false
“Solar Panel Duty on Chinese Imports could Cost UK Billions',” The Guardian, February 19, 2013, https://www.theguardian.com/environment/2013/feb/19/solar-panel-duty-chinese-imports
David M. Hart, “ The Impact of China’s Production Surge on Innovation in the Global Solar Photovoltaics Industry,” ITIF, October 5, 2020, https://itif.org/publications/2020/10/05/impact-chinas-production-surge-innovation-global-solar-photovoltaics
Ibid.
Donald Chung, Kelsey Horowitz, and Parthiv Kurup, “Emerging Opportunities and Challenges in U.S. Solar Manufacturing,” NREL, May 2016, https://www.nrel.gov/docs/fy16osti/65788.pdf
Devon Pendleton, “Elon Musk Tops $500 Billion List of Green Billionaires,” Bloomberg, November 12, 2021, https://www.bloomberg.com/features/2021-green-billionaires/?sref=ZqW0mZJf
For more on Europe’s Green Deal, see here: https://ec.europa.eu/clima/eu-action/climate-strategies-targets/2050-long-term-strategy_en
“UN Secretary-General Issues New Global Roadmap to Secure Clean Energy Access for All by 2030 and Net Zero Emissions by 2050,” SE For All, November 3, 2021, https://www.seforall.org/press-releases/un-secretary-general-issues-new-global-roadmap-to-secure-clean-energy-access-for-all
Ivan Penn, “From 4% to 45%: Energy Department Lays Out Ambitious Blueprint for Solar Power,” The New York Times, September 8, 2021, https://www.nytimes.com/2021/09/08/business/energy-environment/biden-solar-energy-climate-change.html
“Germany Manufacturing Output 1991-2022,” Macrotrends, accessed January 25, 2022, https://www.macrotrends.net/countries/DEU/germany/manufacturing-output
Auke Hoekstra, “Photovoltaic Growth: Reality Versus Projections of the International Energy Agency – With 2018 Update,” Zenmo, January 4, 2019, https://zenmo.com/en/photovoltaic-growth-reality-versus-projections-of-the-international-energy-agency-with-2018-update-2/
Brad Plumer, “Past Climate Treaties Failed. so the Paris Deal Will Try Something Radically Different,” Vox, December 14, 2015, https://www.vox.com/2015/12/14/10105422/paris-climate-deal-history
Patrick Mellor, “Ancient Upheavals Show How to Geoengineer a Stable Climate,” Palladium Magazine, January 28, 2019, https://palladiummag.com/2019/01/28/ancient-upheavals-show-how-to-geoengineer-a-stable-climate/
Samo Burja, “The End of Industrial Society,” Palladium Magazine, March 24, 2021, https://palladiummag.com/2021/03/24/the-end-of-industrial-society/